Neutron scattering brings us a step closer to the quantum computer
A major challenge for future quantum computers is that you have to keep the quantum information long enough to make calculations on it – but the information only has a very short lifespan, often less than a microsecond. Now researchers at the Niels Bohr Institute and the Department of Chemistry at the University of Copenhagen, in collaboration with a team of international researchers, have come closer to a solution. The results are published in the renowned journal Nature Communications.
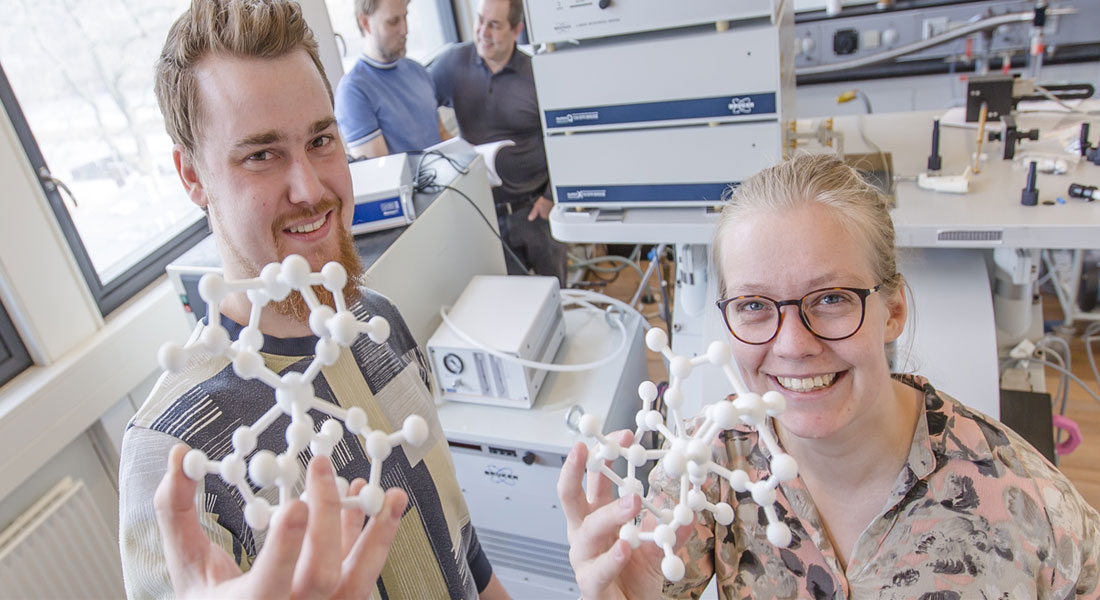
In order to build the quantum computer of the future, you need to be able to store the quantum information – what we call “quantum bits” or “qubits” - (which corresponds to bits and bytes in a traditional computer). Several research groups are experimenting with different ideas for how this can be done in practice.
A team of chemists and physicists from the Niels Bohr Institute and the Department of Chemistry at the University of Copenhagen, as well as collaborators from Germany, France, Switzerland, Spain and the United States, have studied the possibility of producing qubits form organometallic molecules with a single magnetic ion in each molecule.
In these “nanomagnets” there is the particular challenge that random movements in the outside world can interfere with the magnetic ions, so that the quantum information is lost before you can manage to perform calculations with it. Even at ultra-low temperatures just above absolute zero (0.05 Kelvin), where all motion “normally” stops, the system can still be subjected to quantum mechanical disturbances, also known as “tunnelling”.
Mikkel Agerbæk Sørensen, who is the first author of the study, explains that suppressing the tunnelling is considered one of the greatest challenges in the production of new nanomagnets with actual application possibilities: “there are several theoretical models for how to suppress the tunnelling in such molecule-based magnets. With this study, we are the first to have been able to prove the leading model experimentally.”
Changes in the form of the molecule are part of the solution
The researchers have figured out how a combination of chemical synthesis and advanced measurements of energy levels can make very small changes in the form of the molecule, which can suppress the tunnelling. And thus the calculations can be made before the information is lost. To measure the energy levels, the researchers used neutron scattering, which can only be done at major international research facilities – in this case in Munich, Germany and Grenoble, France.
There is still a long way to go to be able to use these nanomagnets in a practical quantum computer, but the researchers have now discovered another “control lever”, namely the geometric form of the molecule that can be used to get closer to the goal. With the construction of the largest neutron facility (ESS) in Lund, Sweden, researchers will have better opportunities to measure and understand tunnelling, thus getting closer to controlling it – and ultimately pave the way for quantum computing.
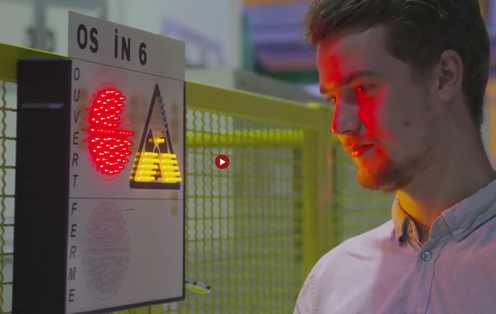
In order to understand the quantum behavior of a molecule-based magnet, it is necessary to measure the energy levels of the molecule very accurately. This is best done with the so-called inelastic neutron scattering. Such experiments can only be done using instruments located at major international research facilities. With the performance of ESS, researchers at the University of Copenhagen will have even better opportunities to conduct such studies. Here Mikkel Agerbæk Sørensen at the entrance of the instrument IN6 at the Institute Laue-Langevin in Grenoble.
The research has been an important part of the PhD studies for Mikkel Agerbæk Sørensen (first author, will defend his PhD dissertation in 2018) and Ursula Bengaard Hansen (defended her PhD dissertation 31/8/2017).
Mikkel Agerbæk Sørensen, Ph.D.-student, Department of Chemistry, University of Copenhagen, Email: mikkel.agerbaek@chem.ku.dk - Homepage: http://www.ki.ku.dk
Ursula Bengård Hansen, Postdoc, X-ray and Neutron Science, Niels Bohr Institute, University of Copenhagen, Email: uhansen@nbi.ku.dk Phone: +45 60 47 86 15
Kim Lefmann, Lektor, X-ray and Neutron Science, Niels Bohr Institutet, University of Copenhagen, Email: lefmann@nbi.ku.dk Phone: +45 29 25 04 76
Jesper Bendix, Professor, Kemisk Institut, University of Copenhagen, Email: bendix@kiku.dk, Phone: +45 35 32 01 01
See also:
Contact
Mikkel Agerbæk Sørensen, Ph.D.-student, Department of Chemistry, University of Copenhagen, Email: mikkel.agerbaek@chem.ku.dk - Homepage: http://www.ki.ku.dk
Ursula Bengård Hansen, Postdoc, X-ray and Neutron Science, Niels Bohr Institute, University of Copenhagen, Email: uhansen@nbi.ku.dk Phone: +45 60 47 86 15
Kim Lefmann, Lektor, X-ray and Neutron Science, Niels Bohr Institute, University of Copenhagen, Email: lefmann@nbi.ku.dk Phone: +45 29 25 04 76
Jesper Bendix, Professor, Kemisk Institut, University of Copenhagen, Email: bendix@kiku.dk, Phone: +45 35 32 01 01