IceCube confirms a 60-year-old prediction from Niels Bohr Institute
Scientists from Niels Bohr Institute are part of an international collaboration observing resonant interactions of high-energy neutrinos in Antarctica. The observation confirms a prediction made by Nobel laureate Sheldon Glashow while working at the Niels Bohr Institute in 1960.
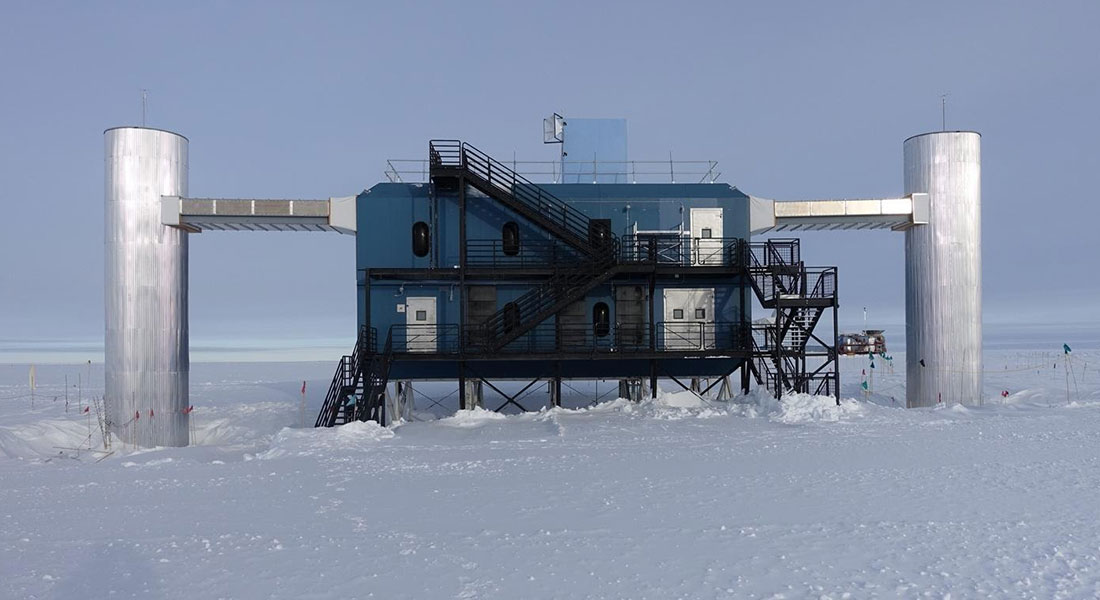
On December 6, 2016, a high-energy particle called an electron antineutrino hurtled to Earth from outer space at close to the speed of light carrying 6.3 petaelectronvolts (PeV) of energy. Deep inside the ice sheet at the South Pole, it smashed into an electron and produced a particle that quickly decayed into a shower of secondary particles. The interaction was captured by a massive telescope buried in the Antarctic glacier, the IceCube Neutrino Observatory.
IceCube had seen a Glashow resonance event, a phenomenon predicted by Nobel laureate physicist Sheldon Glashow in 1960. With this detection, scientists provided another confirmation of the Standard Model of particle physics. It also further demonstrated the ability of IceCube, which detects nearly massless particles called neutrinos using thousands of sensors embedded in the Antarctic ice, to do fundamental physics. The result was published March 11 in Nature.
Sheldon Glashow first proposed this resonance in 1960 when he was a postdoctoral researcher at what is today the Niels Bohr Institute. In a short paper published in Physical Review he predicted that an antineutrino (a neutrino's antimatter twin) could interact with an electron to produce an as-yet undiscovered particle—if the antineutrino had just the right energy—through a process known as resonance.
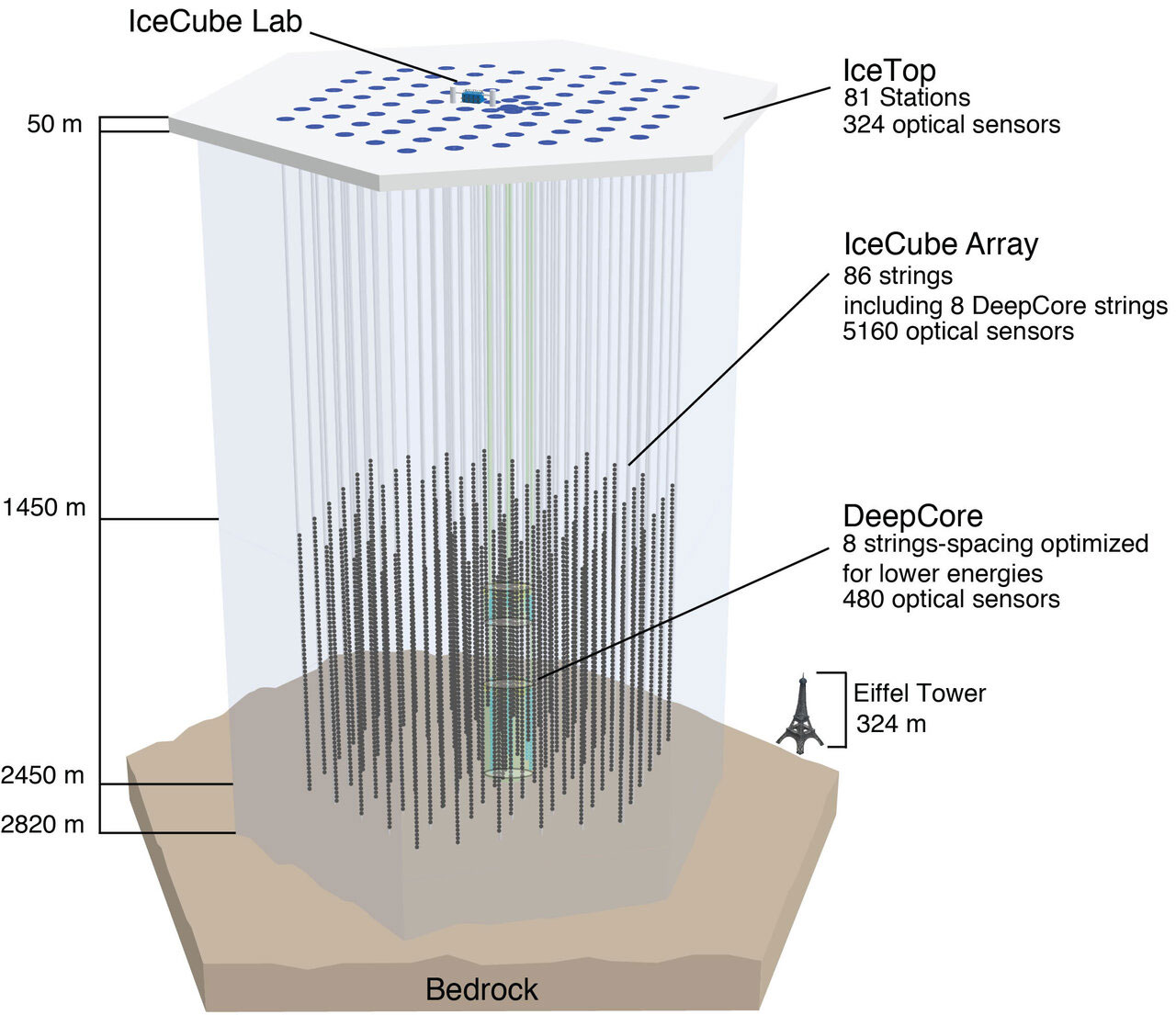
When the proposed particle, the W boson, was finally discovered in 1983, it turned out to be much heavier than what Glashow and his colleagues had expected back in 1960. The Glashow resonance would require a neutrino with an energy of 6.3 PeV, almost 1,000 times more energetic than what CERN's Large Hadron Collider is capable of producing. In fact, no human-made particle accelerator on Earth, current or planned, could create a neutrino with that much energy.
But what about a natural accelerator—in space?
The enormous energies of supermassive black holes at the centers of galaxies and other extreme cosmic events can generate particles with energies impossible to create on Earth. Such a phenomenon was likely responsible for the 6.3 PeV antineutrino that reached IceCube in 2016.
"When Glashow was a postdoc at Niels Bohr, he could never have imagined that his unconventional proposal for producing the W boson would be realized by an antineutrino from a faraway galaxy crashing into Antarctic ice," said Francis Halzen, Gregory Breit and Hilldale Professor at the University of Wisconsin–Madison, the headquarters of IceCube maintenance and operations, and principal investigator of IceCube.
Since IceCube started full operation in May 2011, the observatory has detected hundreds of high-energy astrophysical neutrinos and has produced a number of significant results in particle astrophysics, including the discovery of an astrophysical neutrino flux in 2013 and the first identification of a source of astrophysical neutrinos in 2018. But the Glashow resonance event is especially noteworthy because of its remarkably high energy; it is only the third event detected by IceCube with an energy greater than 5 PeV.
To confirm the detection and make a decisive measurement of the neutrino-to-antineutrino ratio, the IceCube Collaboration wants to see more Glashow resonances. A proposed expansion of the IceCube detector, IceCube-Gen2, would enable the scientists to make such measurements in a statistically significant way. The collaboration recently announced an upgrade of the detector that will be implemented over the next few years, the first step toward IceCube-Gen2.
Glashow, now an emeritus professor of physics at Boston University, echoes the need for more detections of Glashow resonance events. "To be absolutely sure, we should see another such event at the very same energy as the one that was seen," he said. "So far there's one, and someday there will be more."
Last but not least, the result is a triumph of international collaboration. Since 2013, the Niels Bohr Institute is a full member of the IceCube collaboration which includes over 400 scientists, engineers, and staff from 53 institutions in 12 countries. The main analyzers on this paper worked together across Asia, North America, and Europe.
"The connection between a theory developed 60 years ago and this amazing experimental result from IceCube illustrates the potential for new discoveries and observations at the neutrino frontier," says D. Jason Koskinen, Associate Professor and leader of the local IceCube research group at the Niels Bohr Institute.
"The observation of the Glashow resonance proves the great versatility of our detector" says Markus Ahlers, Assistant Professor at the Niels Bohr Institute and IceCube scientist. "We are not only at the forefront of neutrino astronomy but also capable of probing fundamental neutrino properties."
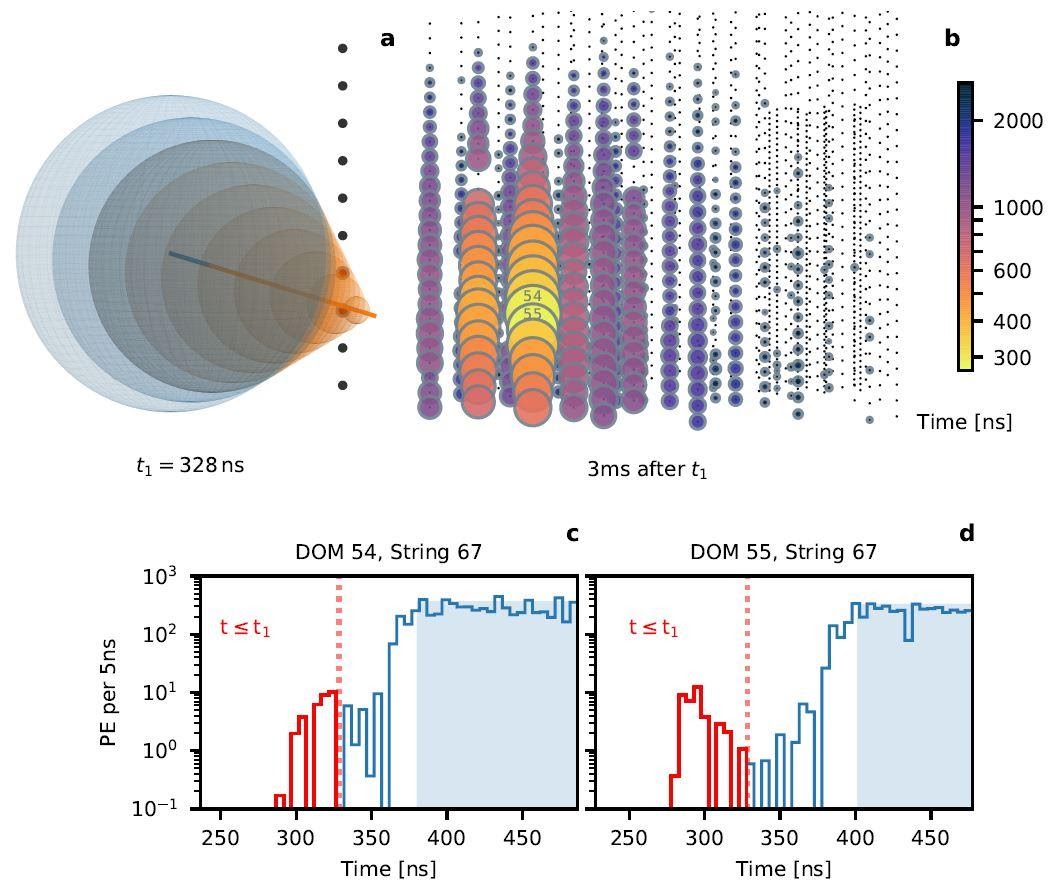
The top left is a schematic of an escaping muon traveling faster than the speed that light propagates in ice, resulting in a cone of Cherenkov light (orange). The muons reach the nearest IceCube sensors (DOMs 54 and 55 on string 67, labeled) ahead of the Cherenkov photons produced by the electromagnetic component of the hadronic shower (blue) since these photons travel at the same speed as light in ice.
The top right is an event view showing sensors that triggered across IceCube. Each bubble represents a sensor; its size is proportional to the deposited charge. Colors indicate the time each sensor first triggered.
The lower panels show distributions of the deposited charge over time on the two earliest hit sensors, DOMs 54 and 55. The dotted red line indicates t1 = 328 ns, the instant shown in the top left of the image. The histogram in red and blue shows photons arriving prior to and after t1, respectively. The blue shaded region denotes saturation of the photomultiplier tube in the sensor
Link to the Article:
“Detection of a particle shower at the Glashow resonance with IceCube,” The IceCube Collaboration: R. Abbasi et al. Nature: https://dx.doi.org/10.1038/s41586-021-03256-1
Topics
See also:
Contact
D. Jason Koskinen, Associate Professor
Niels Bohr Institute, University of Copenhagen
Phone: +45 21 28 90 61
Email: koskinen@nbi.ku.dk
Markus Ahlers, Assistant Professor
Niels Bohr Institute, University of Copenhagen
Phone: +45 35 32 80 89
Email: markus.ahlers@nbi.ku.dk