Multiphoton entanglement using quantum dot spins
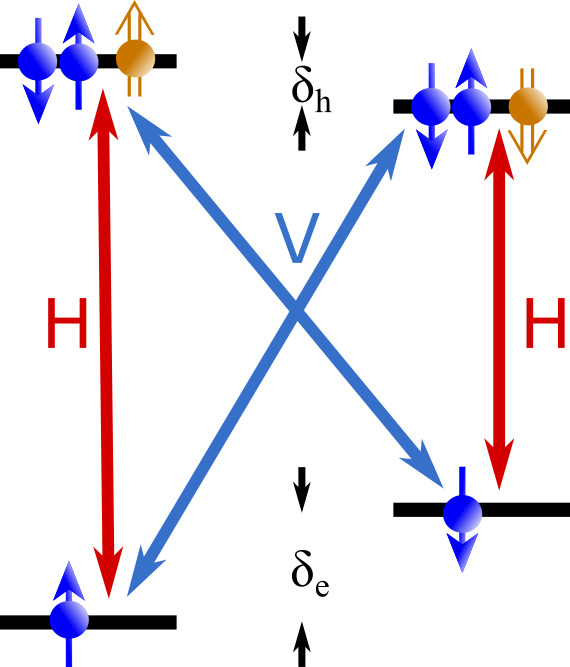
Figure 1. Level diagram of a negatively charged QD in an in-plane magnetic field. This configuration gives a Zeeman splitting of the spin states and allows spin pumping and Raman transitions via the two apparent lamba-systems.
The ability to send quantum information over long distances would allow a number of quantum technologies such as Quantum Key Distribution for secure commination. However, sending information encoded as photons quickly becomes insurmountable over long distances due to photon loss. A possible solution to this problem is the One Way Quantum Repeater: Rather than sending a single photon the entire distance, a so-called cluster state consisting of multiple entangle photons is sent through a chain of repeater stations. At each repeater station the quantum information is transferred onto a “fresh” cluster state, and the gradual photon loss is thus overcome.
Due to their high collection efficiency and photon emission rates, quantum dots (QD) embedded in nanophotonic structures are strong candidates for creating cluster states. By deterministically charging the QD with a single electron or hole and applying a magnetic field, one can realize a spin qubit, see figure 1. This qubit can be initialized, controlled and measured using laser fields [1]. By exciting the QD with a resonant laser pulse, a single photon will be emitted. This photon can be entangled with the QD spin either through its polarization, frequency or emission time. A key goal is then to realize control sequences where the QD is repeatedly excited and manipulated, leading to a long string of photons which are entangled with their neighbor, see figure 2. Beyond emitting photons, a QD spin embedded in a waveguide can modify the waveguide transmission: This can constitute a single spin photon transistor, in which the QD spin state dictates the path of photons guide by the waveguide [2]
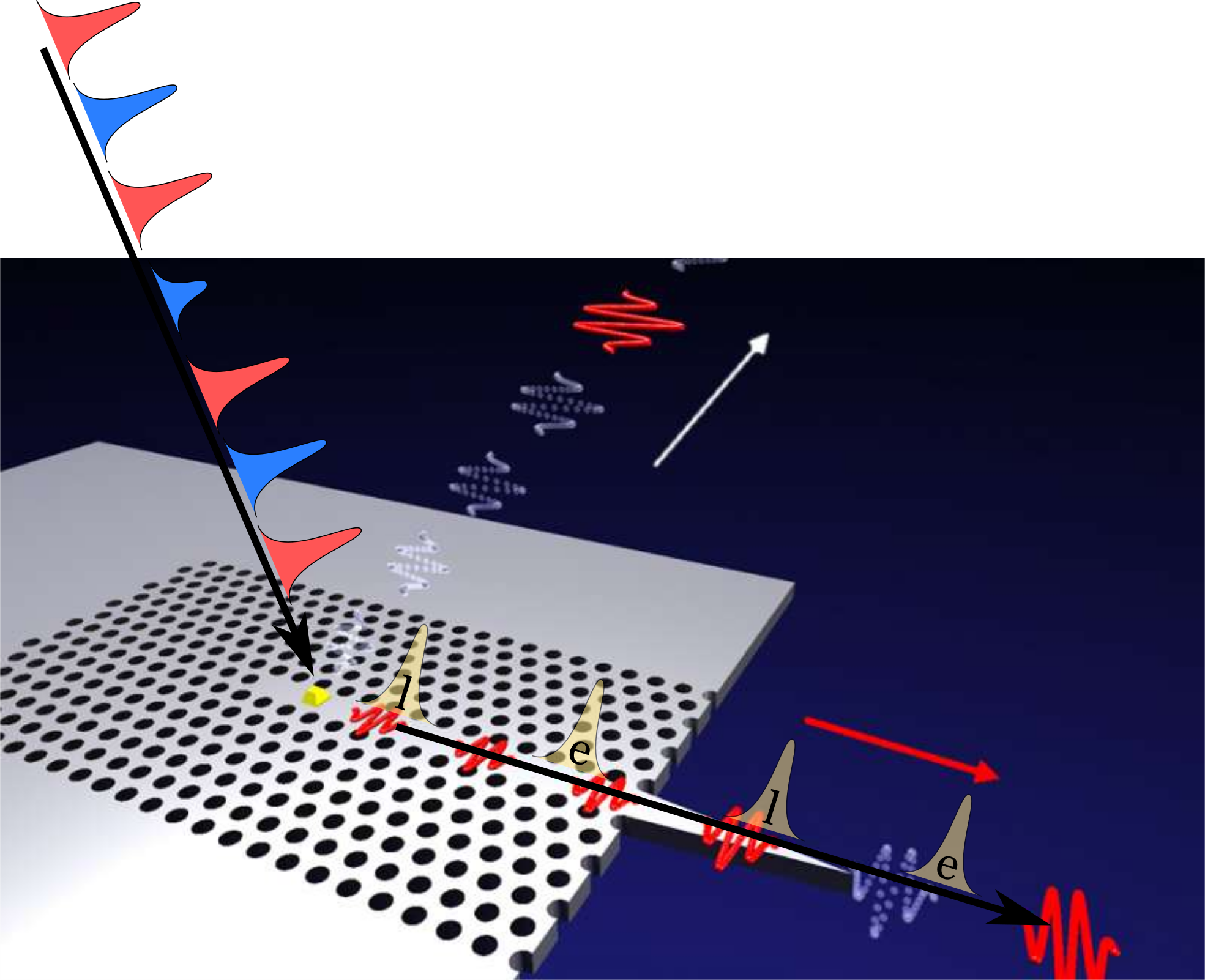
A key challenge of entanglement generation is controlling the QD spin with high fidelity. For instance, the spin must remain coherent during the period over which photons are generated. In this regard the electron spin performs poorly due to its strong coupling to the nuclear spin in the QD, see figure 3. Spin coherence is thus a key area of study, and solutions are emerging where either the nuclear spins are controlled or where the electron spin is replaced by a more coherent hole spin. Another challenge is the nanophotonic structure surrounding the QD. Tailoring this structure allows for highly efficient collection of photons and modifications of the selection rules governing the QD dynamics.
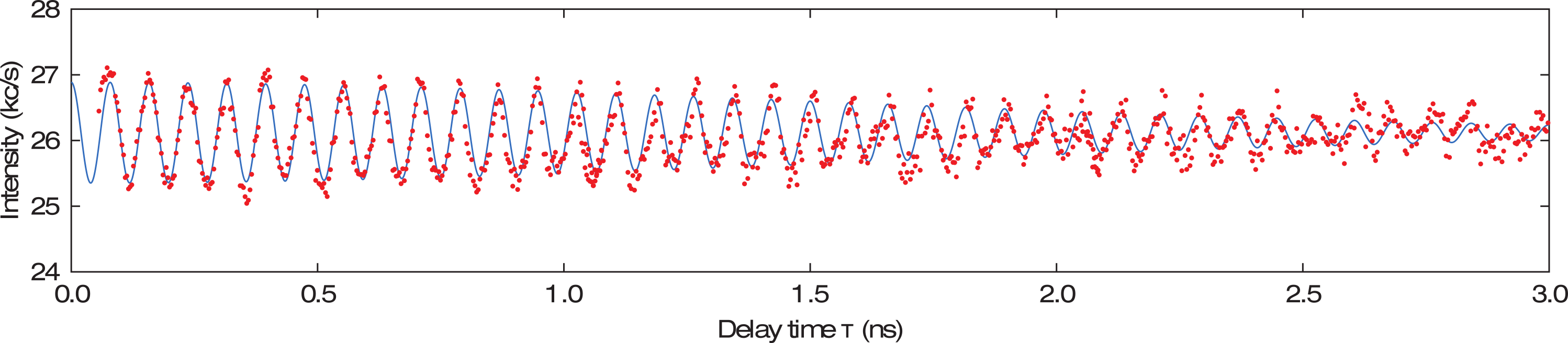