Quantum nonlinear optics
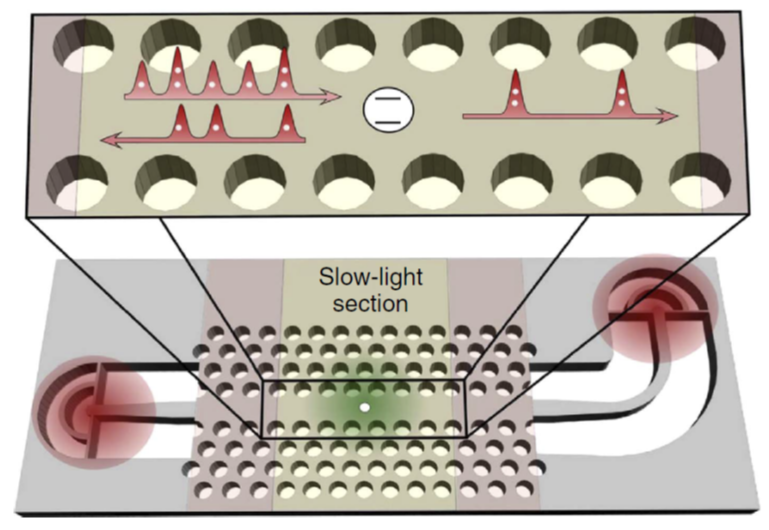
The difficulty in observing true single-photon quantum nonlinearities is that the emitter must couple with near-unity probability to the photonic mode, so that it ‘sees’ every passing photon. Using QDs embedded in photonic-crystal waveguides (see Fig. 1), which both concentrate the light to the region containing the QDs and shield the emitters from the outside world, we have recently observed quantum nonlinear interactions at the single photon level [1]. Excitingly, the nonlinear interaction can be fully coherent, i.e. the phase information of a photon is not lost as it scatters from the QD [2]. A signature of this coherent quantum nonlinearity is the extinction in the transmission of the photon, which arises from the interference of the incident and scattered photon. As shown in Fig. 2, this extinction scales nonlinearly with the number of incident photons interacting with the QD with a critical photon flux of < 1 per emitter lifetime.
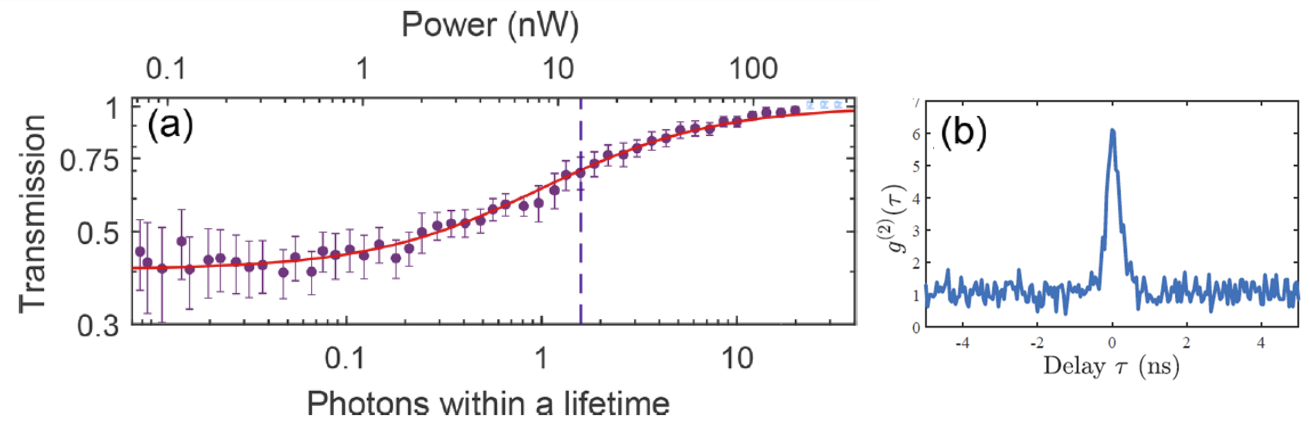
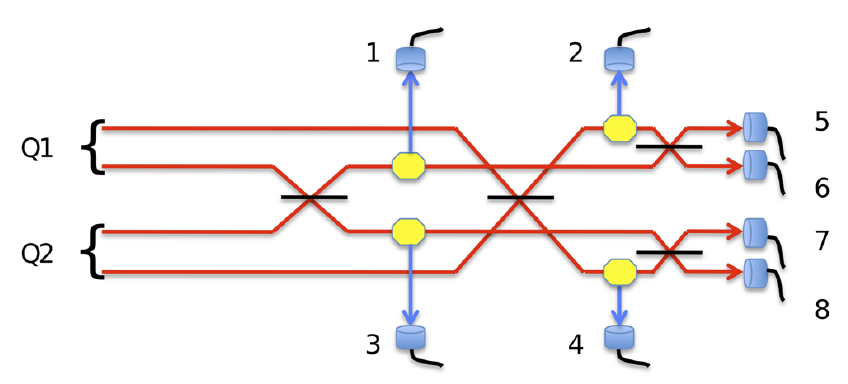
By recording the photon statistics of the transmitted light, the efficiently coupled QD is found to perform photon sorting. By scattering a weak coherent state off the QD, it is found that the single-photon component is effectively reflected, while two and higher-order components are preferentially transmitted. This is revealed by recording the second-order correlation function giving , cf. Fig. 2. Reaching this realm of near-ideal quantum optical nonlinearity raises exciting prospects not only for the fundamental insights into nonlinear light-matter interactions; it also provides a pathway to novel quantum devices. For example, it has been proposed that single-photon nonlinear operations combined with linear optics and photon counting enables constructing a deterministic Bell-state analyzer for photons, cf. the layout in Fig. 3 [3]. This is a fundamental building block required, e.g., for quantum repeaters, a crucial component of a quantum internet.
For additional information and research opportunities, please contact Nir Rotenberg (nir.rotenberg@nbi.ku.dk) or Peter Lodahl (lodahl@nbi.ku.dk).